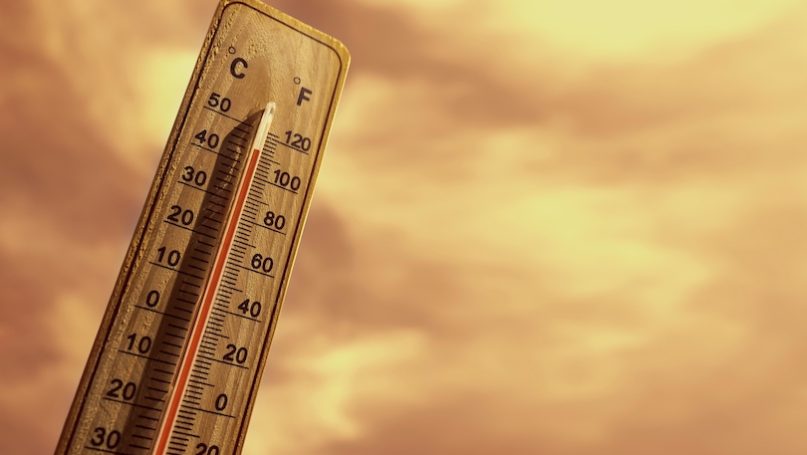
This is an excerpt from Global Climate Justice: Theory and Practice. You can download the book free of charge from E-International Relations. The book version includes all the tables and figures mentioned in this excerpt.
Climate change is happening, and its impacts are increasingly felt by all communities, especially the vulnerable ones that do not have enough resources to adapt to the changes. Global warming, sea-ice melting and sea- level rise have all been accelerating, and with them the frequency and intensity of extreme weather events. Human activities bear most of the responsibility for what we have been observing since the Industrial Revolution. Greenhouse gas emissions must be cut dramatically by about 5-8% every year starting now if we want to achieve net-zero emissions by 2040–2050, and limit global average warming to below 2°C above the pre-industrial level as agreed in Paris in 2015. Failure to act swiftly and effectively will cause even more severe impacts, as indicated by the climate projections generated using state-of-the-art Earth-system models.
This chapter, firstly, presents the evidence for climate change and explains the role of human beings in causing it. Secondly, it explains the concept of an Earth-system model and discusses its role as a tool for understanding the past climate evolution and generating projections of the future climate. Thirdly, it discusses the per capita greenhouse gas emissions of different countries and economic sectors. Finally, it presents climate projections to illustrate how Earth’s climate could evolve under different emissions scenarios.
1. Introduction
The aim of this chapter is to provide a solid, objective and quantitative background to climate change. In Section 2 we will review the greenhouse effect, a key phenomenon that has made Earth a planet where complex lifeforms could evolve, and what are the main greenhouse gases. Understanding the greenhouse effect, the main gases that cause it and its role in the development of life on Earth is essential to be able to recognize what has been happening to Earth’s climate in the 100 years following the Industrial Revolution. Sections 3 and 4 analyse the observed trends in greenhouse gas emissions over time and discuss the observed climate variations and their impacts.
This first part of the chapter will provide a sound understanding of what has been happening to the Earth climate. Section 5 explains what an Earth-system model is and how it can be used to estimate future climate scenarios and their probabilities of occurrence. It discusses how these scenarios are generated and the role that emission assumptions play in determining the future climate. Section 6 presents an analysis of which countries have contributed mostly to the stock of greenhouse gases, and whether there is a clear link between greenhouse gas accumulation in the atmosphere and global warming. Section 7 discusses the quasi-linear relationship between accumulated emissions and average global warming. It also discusses how we must constrain the emissions if we want to limit the global warming below 1.5°C or 2°C degrees. Finally, Section 8 summarizes the key points discussed in this chapter, and discusses the global warming that we could experience in 2050 under four possible emissions scenarios: one with a continuous future increase of greenhouse gas emissions of 1% per year, and three with a continuous future decrease of greenhouse gas emissions by 1%, 3% or 5%.
2. The Greenhouse Effect
The Earth’s temperature would be about 30°C colder if not for the atmosphere’s greenhouse effect. The problem we face today is that too strong a greenhouse effect has been warming the planet in an unprecedented way. And with the warming, we have been experiencing an increased frequency and intensity of extreme weather events and sea-level rise, which have had negative impacts on tens of millions of people who do not have always have sufficient resources to adapt to climate change.
Before discussing climate change and the actions we can take to control it, let us briefly review the greenhouse effect by considering the Earth as a very simple system in equilibrium. The Earth is a system that receives heat in the form of solar radiation and emits heat as a black body. If we apply the first law of thermodynamics (which says that energy must be conserved) to this simple model of the Earth, then there must be an equilibrium between the absorbed and the emitted energy.
The Earth, as any physical ‘body’ that has a temperature above absolute zero (–273.15°C), emits radiation with a certain characteristic (frequency, wavelength, spectrum). The sun, a much warmer body than the Earth, also emits radiation. We can apply the Stefan-Boltzmann law to compute the energy emitted per square meter by a black-body with a temperature T. Here, the term ‘black body’ means that we assume that the Earth and the sun behave as idealized, opaque and non-reflective bodies. The Stefan-Boltzmann law relates the emitted radiation per square meter to the black body’s temperature.
Thanks to the presence of the atmosphere, which absorbs most of the long-wave radiation emitted by Earth’s surface, and re-emits radiation as a black body, the Earth has an average surface temperature of 304°K, which is about 31°C. This temperature (31°C) is about 50°C higher than the temperature the Earth would have without a greenhouse effect.
We should point out that 31°C is higher than the observed average surface temperature, which is about 15°C. This difference is due to the fact that we have assumed that the Earth behaves as a simple system with a very simple atmosphere. In other words, we have over-simplified the Earth and did not consider the complexity of the Earth system. Thus, we should not be surprised if the completed and observed values are different. Despite this, this simple model highlights the important role that an atmosphere has on the Earth’s surface temperature. To make the model slightly more complex, we could assume that the atmosphere is compounded by few different layers instead of only one, with each layer characterized by a different temperature and find a solution for the Earth surface’s temperature that is closer to the observed one.
The atmosphere allows most short-wave solar radiation to reach Earth’s surface, while the amount of the long-wave radiation emitted by the Earth that it absorbs depends on its chemical composition. The principal greenhouse gases are water vapour, carbon dioxide, methane, nitrous oxide and fluorinated gases (Table 1). Note that their concentrations are very small, ranging from about 0.3% (in mass fraction with respect to dry air) for water vapour to much smaller values for carbon dioxide and methane.
There are two key differences between water vapour and the other gases. First, for any air mass, the water vapour concentration strongly depends on the pressure and the temperature of the air. If a mass of air that contains water vapour is cooled and/or compressed, water vapour would condense and precipitate; water continues to cycle, and as a consequence, the total concentration of water vapour in the atmosphere changes very little. Indeed, the time that a molecule of H2O spends in the atmosphere is about 10 days. In the last 100 years, human beings have increased the concentration of carbon dioxide and methane in the atmosphere by more than 50%, from about 270 parts per million (ppm) at the end of the nineteenth century, to about 415 ppm today.
A further, important point to make is that the time that a molecule of CO2 spends in the atmosphere is between 300 and 1,000 years, and the time that a molecule of CH4 spends in the atmosphere is about 10 years. This means that greenhouse gases continue to exercise their effect long after they have been injected in the atmosphere. Because of these two differences, the continuous injection of large quantities of greenhouse gases in the atmosphere has and will for many tens and hundreds of years influence the Earth’s climate.
3. Observed Trends in Greenhouse Gases
The concentration of the greenhouse gases has been increasing since the start of the Industrial Revolution. Figure 1.4 shows the annual mean concentrations of CO2 and CH4, computed using data collected at the Mauna Loa Observatory (Hawaii, United States).
Let us start by looking at CO2. The atmospheric concentration of CO2 has exceeded 400 ppm in 2015. It is worth stressing that the Earth has not seen such a high concentration in the past 2.5 million years (see, e.g., Wallace and Hobbs 2006; Hartmann 2016; IPCC 2021). Note also that the increase follows a roughly exponential curve, and that the annual percent increase has been growing nearly continuously. In fact, as shown in Figure 1.5, while in the 1960s and 1970s the annual percent increase in CO2 was between 0.2-0.4%, in the last two decades it has been above 0.5%. Figure 1.5 also shows that the last two decades have seen a clear positive trend, with the growth rate increasing by about 0.01 every year, from about 0.47% in 2000 to about 0.65% in 2020.
CH4 concentrations have also been increasing from about 1,645 ppb in 1985 (data before 1985 were not available) to 1,879 ppb in 2020 (Figure 1.4). Figure 1.5 shows that the annual growth rate decreased between 1985 and 2000, but since then values have been growing at a fast rate. The last two decades have seen a clear positive trend, with the growth rate increasing by about 0.03 every year, from about 0% in 2000 to 0.60% in 2020.
4. Observed Climate Change
As the concentration of the greenhouse gases increased, the atmosphere has been absorbing more long-wave radiation emitted by the Earth, and thus has been warming. A warmer atmosphere has been emitting more long-wave radiation towards the Earth’s surface, which thus has also been warming. This cause-and-effect mechanism between increasing emissions of greenhouse gases and the global average temperature is a direct consequence of the greenhouse effect that was discussed in Section 1.
Figure 1.6 shows the anomaly of the global annual mean temperature with respect to the pre-industrial value. In other words, for each year, the figure shows the difference between the global annual mean temperature of that year and the global annual-mean temperature of the period 1850-1900. The solid line shows the annual values, while the straight line (which has a slope of ~0.02°C/year) shows the long-term warming trend. Note that superimposed over this linear warming trend of ~0.2°C per decade are natural oscillations of about 0.1-0.2°C. These natural oscillations are due to natural variability, for example linked to large volcanic eruptions or heat exchanged between the Earth’s oceans and atmosphere. In this latter case, the occurrence of large- scale episodes in the tropical Pacific Ocean that causes the ocean temperature to warm (during the El Niño event) or to cool (during La Niña events) produce are natural oscillations in temperature.
Figure 1.6 is based on a new dataset produced by the European Union Copernicus Climate Change Service, the ERA-5 reanalysis (Hersbach et al. 2020), constructed by assimilating all available observations of the Earth system in a state-of-the-art model at the European Centre for Medium-Range Weather Forecasts (ECMWF). It covers the satellite era – the period from 1980 through the present, during which satellite data has allowed scientists to monitor the Earth’s temperature accurately. Figure 1.6 shows that in 2020 the global average temperature was about 1.2°C warmer than the pre-Industrial level, and that the six years from 2015 to 2020 have been the 6 warmest years since 1980.
This warming is not the only evidence that the climate is changing, as was very clearly summarized by the report from the Intergovernmental Panel on Climate Change (IPCC) published in August 2021. The Summary for Policy Makers (SPM) written by IPCC Working Group I confirms that many of the changes observed in the climate are unprecedented in thousands if not hundreds of thousands of years and that some of the changes already set in motion will continue to affect the climate over hundreds to thousands of years in the future (IPCC 2021). It also states, however, that strong and sustained reductions in emissions of CO2 and other greenhouse gases could limit climate change.
The SPM provides updated estimates of the chances of crossing a global warming level of 1.5°C and 2°C (with respect to the pre-Industrial level) in the next decades. These two levels, 1.5°C and 2°C, were agreed by the 196 countries that signed the Paris Agreement in 2015 (UNFCCC 2015) as warming levels that should not be overcome. The SPM confirms that unless there are immediate and large-scale reductions in greenhouse gas emissions, limiting warming to 2°C will be beyond reach. It also confirms that the emissions of greenhouse gases from human activities are responsible for approximately 1.1°C of warming since 1850-1900, and reports that global temperature could reach a warming of between 2°C and 5°C unless immediate and concrete measures are taken to reduce emissions.
These conclusions are not new. They confirm the results published in the IPCC Fifth Assessment Report (AR5) and mentioned in earlier IPCC reports. What is new is that the SPM report of August 2021 is that it includes seven additional years of evidence (AR5 was published in 2014), it reports climate projections based on more realistic and higher resolution models, and it discusses results obtained using new techniques that merge different observations and allow scientists to assess the realism of Earth system models in a more sophisticated way. Furthermore, the report includes results from analyses that combine multi-model projections and thus provide more reliable uncertainty estimates.
It is worth summarizing some of the key impacts of climate change reported in the SPM:
- Climate change is intensifying the water cycle: this brings more intense rainfall and associated flooding, as well as more intense drought in many regions (examples are the floods that affected Central Europe in July 2021, Bangladesh and Australia in June/July 2022, and the forest fires linked to heat waves and droughts that have been affecting many countries during the past two summers of 2021 and 2022).
- Climate change is affecting rainfall patterns: at high latitudes, precipitation is likely to increase, while it is projected to decrease in the Tropics and the Mediterranean region.
- Climate change-induced sea-level rise has accelerated in the past decade, with sea-level rise rates reaching ~3.4mm/year: extreme events related to sea-level rise that previously occurred once every 100 years could happen every year by the end of this century.
- Climate change-induced warming will amplify permafrost thawing, the loss of seasonal snow cover, melting of glaciers (see, e.g., what has been happening in the Alps, including the disaster of the Marmolada glacier in July 2022) and ice sheets and the loss of Arctic Sea ice, which is projected to be ice-free in summer before the end of the century.
- Climate change led to ocean warming, ocean acidification and reduced oxygen levels that have affected ocean ecosystems.
- For cities, some aspects of climate change may be amplified, including heat waves (since urban areas are usually warmer than their surroundings), flooding from heavy precipitation events and sea-level rise in coastal cities.
The SPM also states that without immediate, drastic actions aimed at reducing greenhouse gas emissions, average global warming could reach more than 2.5°C by the end of the century, thus overtaking both warming levels that the 196 countries that signed the Paris Agreement in 2015 (UNFCCC 2015) agreed must not be overcome.
Let us now move to discuss, in the next sections, three key questions that will allow us to understand how we got here and by how much we have to reduce greenhouse gas emissions to limit warming to below 2°C.
- How can we estimate the warming levels we could reach in 10, 20, and 50 years?
- If we look back at the past, which countries have contributed most to greenhouse gas emissions?
- Is there a straightforward link between greenhouse gas emissions and average global warming?
5. Earth-System Models for Climate Studies
Climate projections, such as the ones reported in the IPCC assessment reports, are generated using state-of-the-art models of the Earth system. These models are designed to simulate as accurately as possible all the key processes that determine Earth’s climate. In particular, they aim to simulate the interactions between the atmosphere, the oceans, the cryosphere and land processes. They are based on the laws of physics, such as the conservation of mass and energy and the fact that if a force is applied to a mass of air, it will change its velocity and position. They simulate how clouds are formed, move and cause precipitation. They simulate the interaction between the short-wave solar radiation and the long-wave radiation emitted by the Earth and masses of air in the atmosphere to determine how much energy reaches the Earth surface, how much is absorbed or reflected by the atmosphere. They simulate how air flows pass mountains and how ocean waves affect the presence and/or shape of sea ice. These models see the Earth as a three-dimensional mesh of points with one point every few tens of kilometres on two horizontal axes and every few
hundred metres on a vertical axis (see, e.g., Flato 2011, Gettelmann and Rodd 2016). In total, a model with one grid point every 10 km and with vertical levels every 500 m will have about 109 grid points. At each grid point, the state of the Earth is represented by a set of variables, the so-called state variables. For the atmosphere, for example, these variables are temperature, pressure, wind, specific humidity and cloud types. At the land surface, these variables include information about the type of vegetation and soil, soil temperature and humidity and snow cover. In general, the state variables are chosen so that they are the smallest number of variables that, if they are known, are sufficient to describe in a realistic way the state of the Earth system (e.g., whether it will rain or not, whether intense convective systems will develop or not and whether tropical storms could develop into hurricanes or not). The model aims to represent the state of the Earth system at each grid point at fixed time intervals, say about every 10 minutes.
These Earth system models can be used to predict how the state of the Earth system changes over time, from a few days ahead to years and even decades. The same models are used daily to predict the weather over the next days, weeks and months. To be able to predict the future states of the Earth system, the first step is to know as accurately as possible the state of the system now. This can be estimated by collecting as many observations as possible and by merging them in an objective way that considers their relative accuracy.
To predict the future states of the Earth system, these models, or more precisely the equations that describe how the different model variables interact and change over time, need to be solved. Since the equations are complex and the number of grid points onto which they need to be solved is huge (we said it is about one billion, i.e., 109), they can be solved only numerically, using very fast super-computers. The faster the super-computers, the finer the mesh of the model can be, and the more detailed the description of the physical processes is. In the case of weather prediction, for example, every day more than 600 million observations are collected (95% using instruments on satellites) and about 100 million are assimilated to estimate the current state of the system. It takes between 2–4 hours for all observations to be received; then, the assimilation process (which uses an Earth system model) takes about one hour if one dedicates to it about half of the existing fastest super-computers.
Once the state of the atmosphere has been estimated, the model is integrated numerically on the super-computer and, in about one more hour (again, if one dedicates to this computation about half one of the fastest super-computers), one can generate a forecast valid for the next 10 days. Thus, if one has access to one of the fastest super-computers, in about two hours since all observations have been received (or about six hours since the observations have been collected), one could know the state of the Earth system over the whole globe and know how it will change in the next 10 days.
One of the main differences between making a weather forecast and predicting the climate for the next 10, 20 or 50 years, is the fact that the model has to be integrated numerically for a much longer time. For example, if one wants to generate a climate prediction for the next 50 years with the same model used to make a weather forecast (with a mesh with one grid point every 10 km on the horizontal and every 500 metres on the vertical), one will need about 3 months if they could dedicate to this task half of one of the fastest super-computers. The problem is that although one could dedicate half a super-computer for one hour to the generation of a weather forecast, it is practically impossible to dedicate half of a super-computer for one climate prediction. Thus, more realistically, one should multiply this estimate of 3 months by a factor between 2 and 5, which means that generating a climate prediction for the next 50 years with a mesh with one grid point every 10 km on the horizontal and every 500 metres on the vertical, would take between 0.5 and 2.5 years.
To reduce the time required to complete one climate prediction, one could reduce the resolution from 10 to 50 km on the horizontal, and from 500 to 1,000 metres on the vertical. This reduces the number of grid points by a factor of 50 (5x5x2) and thus makes the whole computation faster by at least that factor, which means that one could generate a climate prediction for the next 50 years in a few weeks. The negative impact of using a reduced resolution is that a climate prediction, compared to a weather prediction, contains less details. Losing these details can have an impact on the capability of a climate prediction to inform us on whether extreme and small- scale events, such as windstorms and flash floods, could become more intense or more frequent in the future.
Despite the fact that Earth system models, when used to make climate predictions, are today characterized by a resolution of about 50 km on the horizontal and 1,000 metres on the vertical, they are still the best tools we have to understand how the climate could change, and what the probabilities that different climate scenarios will occur in the future are. If the models are realistic and simulate future scenarios reliably, they can provide better information than statistics based on the past events, i.e., on the climate of the past decades. This is why many efforts are made by the scientific community to make them increasingly more realistic by including more relevant processes and by improving the simulation of the processes they already simulate.
A second key difference between weather and climate predictions is due to the fact that the climate is very sensitivity to changes in the concentration of the greenhouse gases. Thus, to generate a prediction, one has to assume how the concentration of greenhouse gases will change in the future. In other words, the climate predictions are ‘driven’ by emissions scenarios: high emissions will induce an acceleration of the greenhouse effect and thus an increased warming, while low emissions will slow down the warming rate.
The SPM (IPCC 2021) discusses five possible future climate scenarios, defined by considering a range of possible future developments in anthropogenic drivers of climate change found in the scientific literature. Emissions vary between scenarios depending on socioeconomic assumptions, population growth, levels of climate change mitigation and, for aerosols and non-methane ozone precursors, air pollution controls. Climate projections indicate that, depending on the emissions, the global mean temperature anomaly in 2050 could stay below 1.5°C degrees or increase up to 2.5°C degrees (see IPCC 2021, Figure SPM.8).
The result of these efforts is better knowledge of how the system could behave in the future. For example, the SPM report talks explicitly about the probability that tipping points could be reached and irreversible changes to the Earth system could occur in the future. Tipping points, such as the ones linked to the melting of the Greenland ice sheets or to the deforestation of the Amazon Rainforest, can trigger an acceleration of global warming and are now mentioned more commonly because the scientific community has confidence that the Earth system models used today are capable of simulating how these key Earth system components could realistically evolve in the future.
Let us consider the first question we posed in Section 3: How do we know what level of warming we will reach in 10, 20, and 50 years? The answer is: by integrating state-of-the-art Earth system models and using them to predict possible future scenarios that could occur as a function of assumed greenhouse gas emissions based on an estimated probability that each scenario could occur.
6. Greenhouse Gas Emissions Per Capita Between 1990–2016
Let us now address the second question that was posed in Section 3: If we look back at the past, which countries have contributed mostly to greenhouse gas emissions? We will answer this question by looking at the CO2 emissions data available from the World Bank between 1990 (a year that is often chosen as the reference with respect to which emission reduction targets are set) and 2016 (the last data point available in the World Bank data archive).
We often read that China is the major emitter of CO2, and that Europe contributes to only about 9% of the total emissions. When we read these sentences, often it is not clear whether they refer to the total emissions of a country or a group of countries or to per capita emissions, and it is also not obvious whether they refer to a specific year or to emissions accumulated over a long time period.
Figure 1.7 shows both the total and per capita CO2 emissions the top seven emitters accumulated between 1990–2016 (1990 was chosen since emission reduction targets are often based on a 1990 baseline; 2016 has been chosen since it is the last data point available in the World Bank data). The per capita values have been computed by dividing total emissions by total mid-year population.
In terms of total emissions (Figure 7, left panel), these seven countries (or groups of countries) emitted 68% of the world CO2 emissions during the 1990–2016 period. China and the US contributed the most: 21% and 20% of the CO2 emissions injected into the atmosphere during those 26 years came from these two countries. They are followed by the European Union, which injected about 12% of the world’s total CO2 emissions. The other major emitters followed, with contributions ranging from about 1% (Australia) to about 7% (Russian Federation).
By comparing these values, it should be evident that it is difficult to compare total emissions of countries with different populations, and that one should also consider per capita emissions. It should be noted that emissions per capita are a more ‘just’ measure of emissions since they reflect better whether each person has been given access to the same amount of energy.
The right panel of Figure 1.7 shows the accumulated per capita emissions, for the same period, 1990–2016. Note that now there is a wider difference between the countries. With respect to this measure, one person living in the US has emitted 500 tonnes of CO2 over those 26 years, compared to about 120 tonnes for a person living in China. Australia, Canada and Russia now rank second, third and fourth among the major emitters. Note that an average person living in India has emitted only 30 tonnes of CO2 in 26 years, about the same amount that persons living in the top four polluters injected in the atmosphere in just two years.
It is interesting to compare these numbers with the world average CO2 emissions per capita – 115 tonnes: note that a person living in the top four polluters emitted about four times more than the world average, a person living in the European Union about two times more, a person living in China the same as the average and a person living in India four times less.
As economies developed and transformed throughout the years, the emission ranking changed. Figure 1.8 gives a snapshot of the most recent year, 2016, for which the data are available in the World Bank database. It shows that, in 2016 and in terms of total emissions, China is the top contributor with a contribution of about 29% of the world emissions, followed by the US with about 15% and the European Union with about 8.5%. But if we look at emissions per capita, the US, Canada and Australia remains the top polluters, with emissions per capita of about 15 tonnes in 2016, compared to about seven tonnes for a person living in China, 6.5 tonnes for a person living in the European Union and 1.8 tonnes for a person living in India. In 2016, the world average CO2 emissions per capita was about 4.5 tonnes. Thus, it is evident that there are still countries that emit about four times more than the average and countries that emit about four times less.
Let us consider the second question posed in Section 3: If we look back at the past, which countries have contributed most to greenhouse gas emissions? The answer depends on whether we use total or per capita emissions. China, the US and the European Union have contributed the most if we look at total emissions accumulated between 1990–2016. By contrast, if we look at per capita emissions accumulated between 1990–2016, the top emitters are the US, Australia, Canada and Russia, which emitted twice as much as an average person living in the European Union and four times as much as a person living in China.
7. Link between Greenhouse Gases and Global Warming
Let us know address the third of the three questions posed in Section 3: Is there a straightforward link between greenhouse gas emissions and average global warming? The latest IPCC report (IPCC 2021) talks about a quasi-linear relationship between the amount of greenhouse gases that are released into the atmosphere and global warming. Indeed, if we contrast global warming, measured by the global annual average surface temperature anomaly from 1980 until the present (Figure 1.6) against the greenhouse gases accumulated since 1980 (Figure 1.9), we can detect a quasi-linear trend.
The fitted straight line shown in Figure 1.9 has a slope of 0.5°C/1,000 Gt, which means that during this period, each additional 1,000 Gt of greenhouse gases in the atmosphere has led to 0.5°C of warming on average. Note that the coefficient of determination of the linear fit, R2 = 0.82, confirms the existence of a robust linear relationship. Note that there are variations around this linear relationship, reflecting the fact that each year’s climate is influenced not only by greenhouse gas concentrations, but also by atmosphere and ocean dynamics (e.g., whether the year was characterized by a strong El Niño or La Niña event and whether other changes in the large- scale circulation caused heat waves over large areas of the globe).
The SPM talks about a slope of about 0.45°C/1,000 Gt, which is close to the relationship we found. We can use this quasi-liner relationship to estimate how much we could emit to limit warming. For example, given that in 2018 the average global warming was 1.1°C above pre-Industrial levels, we can only emit about 2,000 Gt more of greenhouse gases if we want to keep warming below 2°C. In 2019, we emitted about 45 Gt of greenhouse gases (of which ~30 Gt was CO2 and ~15 Gt was CH4 and other greenhouse gases). If we continue to emit, on average, about 45 Gt per year, in about 45 years we would reach that amount. If instead we want to keep global warming below 1.5°C, we have to limit emissions to less than 900 Gt. If we continue to emit as we did in 2019, i.e., about 45 Gt/year, we will surpass that value by 2038.
Let us consider the third of the three questions posed in Section 3: Is there a straightforward link between greenhouse gas emissions and average global warming? The answer is yes: since there is a quasi-linear relationship between accumulated emissions and global average warming, we can estimate in a straightforward way how the climate will look like in the future.
8. Concluding Remarks
We started our discussion in Section 1 by examining the greenhouse effect and the key role it has played in the development of complex lifeforms on planet Earth. Then, in Section 2, we discussed the observed, continued acceleration of increasing concentrations of greenhouse gases in the atmosphere. This acceleration is mainly due to human activities, as stated in the latest SPM report (IPCC 2021, see also Fig. SPM.1): ‘It is unequivocal that human influence has warmed the atmosphere, ocean and land. Widespread and rapid changes in the atmosphere, ocean, cryosphere and biosphere have occurred’.
In Section 3, we documented the impact of the continued increase in greenhouse gas concentrations on the average global surface temperature between 1980 and 2020. We also briefly discussed some uncontroversial impacts of climate change, e.g., sea-level rise, sea-ice extension (especially in the Arctic) and increasingly intense and frequent extreme events.
In Section 4, we summarized the state of the climate, and discussed what has been happening to the global surface temperature since the Industrial revolution, and the impact of global warming on sea-level rise, the melting of sea-ice and glaciers and extreme weather events.
We then posed three key questions:
- How can we estimate the warming levels we could reach in 10, 20, and 50 years?
- If we look back at the past, which countries have contributed most to greenhouse gas emissions?
- Is there a straightforward link between greenhouse gas emissions and average global warming?
In Section 5, we addressed the first question and found that Earth system models are the best tools we have to predict future scenarios and their probabilities of occurrence. We have illustrated what an Earth system model is, the fact that they are based on the laws of physics and how they can be integrated numerically on super-computers to provide us with reliable information about the future climate.
In Section 6, we addressed the second question and found that the answer depends on how we measure emissions and whether emissions are accumulated over a long time period (in our case, we accumulated the emission over the 26 years, between 1990–2016) or over a short time period, such as the year 2016. We also said that the answer depends on whether one compares countries’ and groups-of-countries’ total emissions or their per capita emissions. We showed that if one looks at per capita emissions between 1990–2016, the ranking sees the US as the top contributor to climate change, followed by Australia, Canada, the Russian Federation, the European Union and then China.
In Section 7, we discussed the third question and showed that there is a quasi-linear relationship between greenhouse gas emissions and global warming. This relationship was also pointed out explicitly in the SPM report of 2021. Looking at data from 1980 to 2020, we showed that each additional 1,000 Gt of greenhouse gas emissions led 0.5°C of warming on average. This relationship can be used to make a simple, zero-dimensional prediction of the future climate. If we apply it, considering that the world injected about 45 Gt of greenhouse gases into the atmosphere in 2019, and if we assume that emissions will continue at this level on average for the next few decades, we can predict that in about 13 years the warming will be about 1.5°C warmer on average than it was before the Industrial Revolution. We can also estimate that in about 40 years the rate of global warming will be about 2°C.
It should now be clear what has been happening to the climate, and why it is necessary to start immediately reducing emissions of greenhouse gases. If we want to keep warming below 2°C, we have to reduce the emissions substantially – by 5-8% per year on average, until we reach net-zero emissions in 2050. We can apply this simple quasi-linear relationship between greenhouse gas emissions and average global warming to look ahead into the future. If we look at the last 10 years, annual emissions grew from 39.8 Gt in 2009 to 45.8 Gt in 2018 (data from the World Bank).
Figure 1.10 shows, with the black diamonds, total greenhouse gas emissions and projected warming in 2050 computed applying the linear relationship discussed in Section 6, under four different emission scenarios:
- A continued average increase of 1% a year, as was the case between 2009–2018: this would cause a further 1,738 Gt of greenhouse gases to be injected into the atmosphere, which would reach 3,173 Gt and a warming of 2°C in 2050;
- A continued average decrease of 1% a year, starting from 2019: this would cause a further 1,249 Gt of greenhouse gases to be injected into the atmosphere, which would reach 2,684 Gt and a warming of 1.75°C in 2050;
- A continued average decrease of 3% a year, starting from 2019: this would cause a further 9994 Gt of greenhouse gases to be injected into the atmosphere, which would reach 2,429 Gt and a warming of 1.61°C in 2050;
- A continued average decrease of 5% a year, starting from 2019: this would cause a further 703 Gt of greenhouse gases to be injected into the atmosphere, which would reach 2,138 Gt and a warming of 1.47°C in 2050.
Thus, only by decreasing the emissions on average by ≥5% every year can we limit average global warming to below 1.5°C above pre-Industrial levels. But it is worth mentioning that even in this scenario, we will still be injecting about 9 Gt of greenhouse gases into the atmosphere every year by 2050. If we want to achieve ‘almost’ net-zero emissions, we must reduce average emissions by ≥8% every year: an average annual reduction of 8% a year would lower the emissions from the 45 Gt emitted in 2019, to about 3 Gt in 2050.
References
Flato, G. M. 2011: Earth system models: an overview. WIRE’s Climate Change, 2: 6. pp. 783–800 https://www.doi.org/10.1002/wcc.148
Gettelmann, A. and Rodd, R.B. 2016. Demystifying climate models. A User Guide to Earth System Models. Berlin: Springer.
Hartmann, D. L. 2016: Global Physical Climatology. Second edition. Amsterdam: Elsevier.
Hersbach, H., Bell, B., Berrisford, P., Hirahara, S., Horanyi, A., Munoz- Sabater, J., Nicolas, J., Peubey, C., Radu, R., Schepers, D., Simmons, A., Soci, C., Abdalla, S., Abellan, X., Balsamo, G., Bechtond, P., Biavati, G., Bonavita, M., De Chiara, G., Dahlgren, P., Dee, D., Diamantakis, M., Dragani, R., Flemming, J., Forbes, R., Fuentes, M., Geer, A., Haimberger, L., Healy, S., Hogan, R. J., Holm, E., Janiskove, M., Keeley, S., Laloyaux, P., Lopez, P., Lupu, C., Radnoti, G., de Rosnay, P., Rozum, I., Vamborg, F., Villaume, S., Thepaut, J.-N., 2020: The ERA5 global reanalysis. Q. J. Roy. Meteorol. Soc., 146, 1999–2049. https://www.doi.org/10.1002/qj.3830
IPCC, 2021: Summary for Policymakers. In: Climate Change 2021: The Physical Science Basis. Contribution of Working Group I to the Sixth Assessment Report of the Intergovernmental Panel on Climate Change [Masson- Delmotte, V., P. Zhai, A. Pirani, S.L. Connors, C. Péan, S. Berger,
N. Caud, Y. Chen, L. Goldfarb, M.I. Gomis, M. Huang, K. Leitzell, E. Lonnoy,
J.B.R. Matthews, T.K. Maycock, T. Waterfield, O. Yelekçi, R. Yu, and B. Zhou (eds.)]. Cambridge University Press.
United Nations Framework Convention on Climate Change (UNFCCC) 2015; The Paris Agreement. Available from the UNFCCC web site: https://unfccc.int/ sites/default/files/english_paris_agreement.pdf
Wallace, J. M. and Hobbs, P. V. 2006: Atmospheric Science – An introductory survey. Second Edition. Amsterdam: Academic Press.
Further Reading on E-International Relations
- Opinion – Post-COVID-19 Climate Change Politics
- Has Climate Change Ended Nature?
- From Rio to Paris: International Climate Change Treaties Between Consensus and Efficacy
- Agroecology, Climate Change Induced Polycrisis and the Transformation of Food Systems
- Taking Climate Change Seriously: The ‘Values Approach’
- Reflecting on Democracy, Corruption and Climate Change in the COVID-19 Era